Engineers explore environmental concerns of nanotechnology
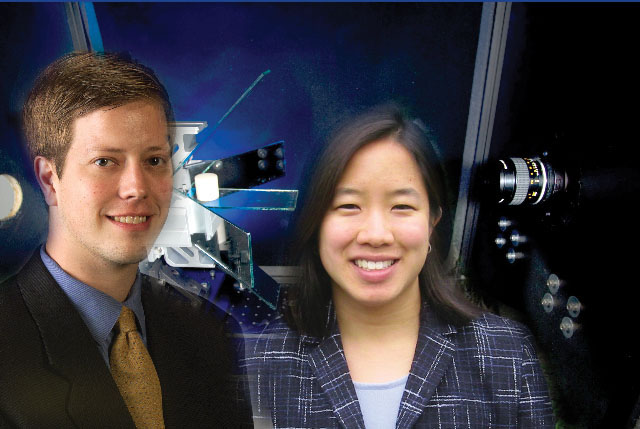
As researchers around the world hasten to employ nanotechnology to improve production methods for its various applications that range from manufacturing materials to creating new pharmaceutical drugs, a separate but equally compelling challenge exists.
History has shown that previous industrial revolutions, such as those involving asbestos and chloroflurocarbons, have had some serious environmental impacts. Might nanotechnology also pose a risk?
Linsey Marr and Peter Vikesland, two faculty members in the Via Department of Civil and Environmental Engineering, are among the ground-breaking investigators in this field. They are part of the national Center for the Environmental Implications of NanoTechnology (CEINT), funded by the National Science Foundation (NSF) in 2008. Along with Michael Hochella, University Distinguished Professor of Geosciences, they represent Virginia Tech’s efforts in a nine-member consortium awarded $14 million over five years, starting in 2008. Virginia Tech’s portion is $1.75 million.
CEINT is dedicated to elucidating the relationship between a vast array of nanomaterials — from natural, to manufactured, to those produced incidentally by human activities — and their potential environmental exposure, biological effects, and ecological consequences. It will focus on the fate and transport of natural and manufactured nanomaterials in ecosystems.
Headquartered at Duke University, CEINT is a collaboration between Duke, Carnegie Mellon University, Howard University, and Virginia Tech as the core members, as well as investigators from the University of Kentucky and Stanford University. CEINT academic collaborations in the United States also include on-going activities coordinated with faculty at Clemson, North Carolina State, University of California at Los Angeles, and Purdue universities. At Virginia Tech, CEINT is part of the university’s Institute for Critical Technology and Applied Science.
Scientists and engineers at the center have outlined plans to conduct research on the possible environmental health impacts of nanomaterials. The plans include new approaches, such as creating a predictive toxicology model based on cell assays and building ecosystems to track nanoparticles.
Characterization of airborne particles
In one of the novel ways Marr is conducting her tests, she and her colleagues are growing human lung cells and placing them in chambers that leave the lung cell surface exposed to air. This placement allows for direct contact of the cells with aerosolized particles at the air-liquid interface (ALI). One of Marr’s post-doctoral researchers, Amara Holder, and colleagues from Berkeley have previously exposed the cells to particles in diesel exhaust and a methane flame. They compared the ALI exposure to conventional in vitro exposure, where particles are suspended in a liquid cell culture medium.
“Our findings showed the ALI exposure inhalation route is a relevant in vitro approach and is more responsive than the conventional exposure to particle suspensions,” they concluded. Now, Marr and her colleagues are repeating the exposure with engineered nanoparticles. The researchers will enhance the deposition of smaller particles by generating an electric field and “relying on the electrophoretic force to drive charged particles to the cell surface.”
“With this design, lung cells can be exposed to substantial numbers of aerosolized engineered nanoparticles, such as silver and metal oxides, as single particles rather than large agglomerates,” Marr explained. A challenge in tests of nanoparticles’ toxicity has been that very small particles like to form aggregates, so testing interactions of the smallest particles with cells requires special approaches.
Marr and one of her graduate students, Andrea Tiwari, have selected the C60 fullerene as a model for carbonaceous nanomaterials because of its relative simplicity, evidence of toxicity and rich history in the scientific literature. The discovery of the C60 compound in 1985 earned Harold Kroto, James R. Heath, and Richard Smalley the 1996 Nobel Prize in Chemistry. C60 fullerenes and variations on them are being used throughout the nanotechnology industry.
“Airborne carbonaceous nanomaterials are likely to be found in production facilities and in ambient air and may exhibit toxic effects if inhaled,” Marr and Tiwari said. They further theorized that when exposed to the air, nanomaterials are likely to be chemically transformed after the exposure to oxidants in the atmosphere.
In their preliminary studies, results indicate that “oxidation does impact solubility, as absorbance after resuspending in water is lower for fullerenes exposed to ozone.” The implication is that reactions in the atmosphere can transform nanoparticles and make them more likely to dissolve in water once they deposit back to earth. There, they can travel farther and come in contact with more organisms than if they were stuck to soil.
To collect airborne nanoparticles for analysis, Marr’s group designed a low-cost thermophoretic precipitator that uses ice water as a cooling source and a 10-W resistor as the heating source. They flowed synthetic aerosols through the precipitator and used a transmission electron microscope to inspect the particles.
“Preliminary analysis confirmed that this precipitator was effective in collecting nanoparticles of a wide range of sizes and will be effective in future studies of airborne nanoparticles,” Marr said.
As her work in this field progresses, Marr was able to use her research in the characterization of airborne particle concentrations during the production of carbonaceous nanomaterials, such as fullerenes and carbon nanotubes, in a commercial nanotechnology facility. Based on the measurements of her study, done with Behnoush Yeganeh, Christy Kull, and Mathew Hull, all graduate students, they concluded that engineering controls at the facility “appear to be effective in limiting exposure to nanomaterials,” and reported their findings in the American Chemical Society’s publication Environmental Science and Technology (Vol. 42, No. 12, 2008)
However, they point to the limitations of this initial study that focused mainly on the physical characterization, and which did not differentiate between particles generated by nanomaterial soot production and those from other sources.
Effects of carboxylic acids on nC60 aggregate formation
“The increasing production and application of the C60 fullerene due to its distinctive properties will inevitably lead to its release into the environment,” Marr’s colleague, Vikesland, said. Already, the biomedical, optoelectronics, sensors and cosmetics industries are among the users of the C60 fullerene.
“Little is currently known about the interaction of the C60 fullerene with the constituents of natural waters, and thus it is hard to predict the fate of C60 that is released into the natural environment,” Vikesland added. “The C60 fullerene is virtually insoluble in water.”
However, one of the components of natural water is natural organic matter (NOM). When the C60 fullerene is released in water, it forms “highly stable dispersed colloidal C60 aggregates or nC60,” Vikesland explained. These aggregates can exhibit significant disparities in aggregate structure, size, morphology, and surface charge and behave very differently than the C60 alone.
The problem with NOM is its randomness, resulting in diverse characteristics of the aggregates that form when they mix with the C60.
So, Vikesland is looking at small molecular weight carboxylic acids such as acetic acid, tartaric acid, and citric acid, all widely detected constituents of natural water and biological fluids. He and his graduate student Xiaojun Chang of Blacksburg, Va., have specifically looked at the formation of nC60 in acetic acid (vinegar) solutions, subjected the aggregates to extended mixing, and found that the solution’s chemistry differs substantially from nC60 mixed in water alone.
“The citrate affects the formation of the nC60 in two ways,” Vikesland said. It alters the pH, a key factor in controlling the surface charge of nC60 and it directly interacts with the C60 surface.
Vikesland explained the significance of this result. When nC60 is produced in the presence of the carboxylic acids, its aggregates differ significantly from those produced without the acids. In general, Vikesland said, these aggregates have more negative surface charges and are more homogenous than those produced in water alone.
“These results suggest that the ultimate fate of C60 in aqueous environments is likely to be significantly affected by the quantities and types of carboxylic acids present in natural systems and by the solution pH,” Vikesland added. Furthermore, because carboxylic acids are common in biological fluids, Vikesland is interested in how his findings relate to the mechanisms by which C60 interact with cells in vivo.
These acids may significantly affect any conclusions ultimately reached regarding the impact of the C60 fullerene into the environment. His current work appears in an issue of Environmental Pollution 157 (2009), pp. 1072-1080.