Ancient rocks may bring dark matter to light
With new imaging capabilities, the first successful dark-matter detector might be some old rock.
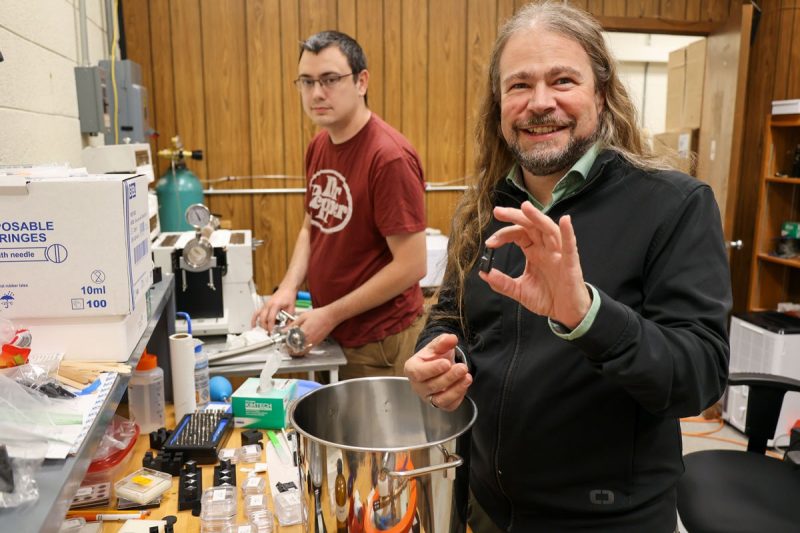
The visible universe — all the potatoes, gas giants, steamy romance novels, black holes, questionable tattoos, and overwritten sentences — accounts for only 5 percent of the cosmos.
A Virginia Tech-led team is hunting for the rest of it, not with telescopes or particle colliders, but by scrutinizing billion-year-old rocks for traces of dark matter.
In leading a transdisciplinary team from multiple universities on this unconventional search, physics’ Patrick Huber is also taking an unconventional step: from theoretical work into experimental work.
With support from a $3.5 million Growing Convergence Research award from the National Science Foundation and a separate $750,000 award from the National Nuclear Security Administration, Huber is building a new lab in Robeson Hall to test dark matter theories — and see what else might come to light along the way.
Dark matter is super dark
Scientists can only infer dark matter’s existence because objects in the universe fall faster than they should around the center of galaxies. Gravity from this unseen substance accounts for the extra oomph.
Unlike the bump and grind of regular stuff, dark matter is thought to interact only very weakly with other matter, imperceptible except when one happens to bump into a nucleus of a visible matter atom. Recoiling from the collision like an atomic billiard ball, the nucleus deposits a spark of energy.
Over the past 50 years, physicists have conducted all manner of dark-matter experiments in hopes of witnessing one of these rare recoil events.
So far? Dark matter has stayed dark. Physicists haven’t turned up any hard evidence for dark matter. Now they’re turning down — deep down.
Paleodetectives
If dark matter exists, there’s a chance it has interacted with the Earth at some point in its 4.6 billion-year-old history. What if, instead of waiting for dark matter to come to them, scientists could excavate ancient evidence from minerals deep in the Earth?
While the idea for using rocks as subterranean detectors was first proposed in the 1980s, technological advances prompted researchers, including Huber, to revisit this idea.
“It’s crazy. When I first heard about this idea, I was like — this is insane. I want to do it,” said Huber, the William E. Hassinger, Jr. Senior Faculty Fellow.
Huber, being a theoretical physicist, came up with a theory of how to solve it. But the theory wasn’t enough. If this plan was possible, he wanted to see what it would take to execute it.
“Other people in their midlife crisis might take a mistress or get a sports car. I got a lab,” Huber said.
Who knocked the nuclei?
By developing and using sophisticated imaging techniques, Huber and his collaborators hope to uncover miniature trails of destruction left by long-ago dark matter interactions inside crystal lattice structures.
When a high-energy particle bounces off a nucleus inside a rock, the explosive recoil can pop a nucleus out of place, said Vsevolod Ivanov, a researcher at the Virginia Tech National Security Institute who is collaborating with Huber. The ejected nucleus and the empty gap it leaves behind represent structural changes within crystal.
“We’ll take a crystal that’s been exposed to different particles for millions of years and subtract the distributions that correspond to things we do know,” Ivanov said. “Whatever is left must be something new, and that could be the dark matter.”
Most dark matter experiments are conducted underground to cut back on interference from other high-energy particles called cosmic rays, but going underground presents a new set of problems. The planet pulses with a radioactive background that can also jostle nuclei. University Distinguished Professor Robert Bodnar, recently inducted into the National Academy of Sciences, will be working with Huber’s team to identify, locate, and characterize minerals that could serve as suitable detectors.
Proof in 3D
To start in on this massive imaging task, Huber is working with researchers at the University of Zurich’s Brain Research Institute who provided access to special microbiology imaging technology typically used to image animal nervous systems.
The team has already started generating 3D renderings of high-energy particle tracks in synthetic lithium fluoride. This artificial crystal won’t make a good dark-matter detector, said Huber, but it will help establish the full range of signals while keeping the crystal intact. In an unexpected twist, applications of lithium fluoride imaging technology include “nuclear transparency devices,” which might look like backpack-sized monitoring devices for nuclear reactors.
With tangential outputs from this “insane” research objective already proving of immediate value, Huber his collaborators will dig deeper and look closer to see if an old rock can tell us how the stars fly around the galaxy.