Pinar Acar receives NSF CAREER award to design the materials of the future
Acar’s team specializes in creating digital profiles of materials. In this project, the team will be creating digital models set to music.
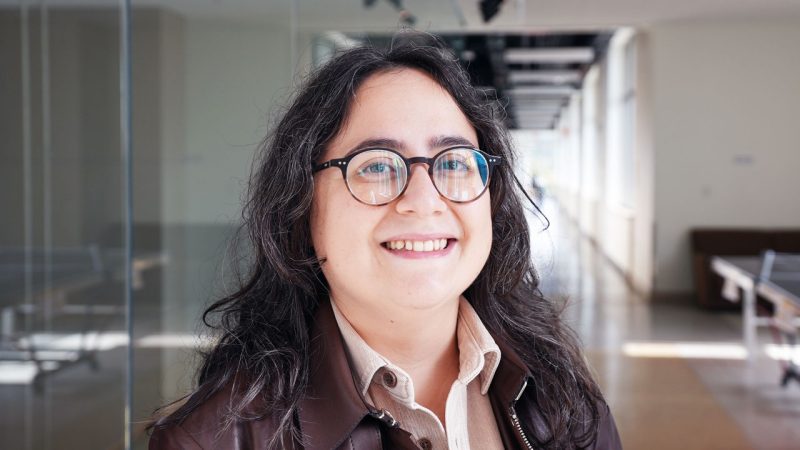
Pinar Acar, assistant professor in the Department of Mechanical Engineering, has been granted a $549,365 National Science Foundation Faculty Early Career Development (CAREER) award to develop a database of profiles that will equip manufacturers with powerful tools to choose a metamaterial that best serves their needs. With that same digital information, the team also will convert the data into music.
The CAREER award is the most prestigious award given by the foundation for early career faculty, encouraging them to serve as academic role models in research and education and to lead advances in the mission of their organizations.
Decoding material manufacturing
When materials are manufactured, two factors come into play: the substance from which the material is made and the microscale architecture that holds it together. Both factors are important. Innovative manufacturers often find themselves searching for ways to produce materials that are lighter, more durable, or lower in cost. Determining the best way to achieve those goals and finding an ideal material can be difficult.
Manufacturers might choose a substance that is extremely durable, but a poor architecture could undermine that strength. Conversely, a substance that is less strong could be given an architecture with strength beyond its raw capabilities. The substance's ability to twist, expand, contract, and stretch under strain can also be taken into account, and any of those properties might create a more ideal fit in the overall design.
Engineering the microscopic architecture offers myriad options because the properties of a substance perform differently depending on the shapes and patterns chosen. Architecture with a square pattern gives different properties than hexagons and triangles, and stacking different layers from varying shapes might create even more unique properties.
Material plus architecture equals topology, and finding the magic combination of a perfect topology is a job for Acar’s speciality: mathematical modeling.
“I consider myself more of a design person than a materials person,” Acar said. “There is lots of research going on in topology, but most of the applications are quite limited. Most of the computational studies are two-dimensional. What I propose here is overcoming those barriers.”
Recoding in forward and inverse
Understanding how materials behave is the first step in forming an optimal material topology. Applying the materials for specific uses is the second. In Acar’s view, researchers might approach the topic from one of two directions: either forward or inverse.
“Forward means maximizing or minimizing existing material properties,” Acar said. “Inverse means we are trying to achieve a target value. When we have a value for something like toughness, I can find the corresponding topology. By solving the inverse problem, I can tell you what underlying architecture, or topology, can provide those properties.”
This project will combine both the forward and inverse. Acar’s previous research background includes using data-driven mathematical models to solve complex problems with material design. By pairing the forward method of using known material properties with her familiar territory in mathematically modeled microscale architecture design combinations, Acar’s team will build a database that answers questions about material applications that don’t yet exist.
“Inverting almost always produces multiple solutions,” said Acar. “There is never one single design that gives you the desired outcome. We are also interested in finding the other designs that are equal in terms of their mechanical properties.”
While Acar’s expertise with mathematical modeling generally places her work in the realm of the theoretical, testing physical materials also will be part of this study. The early stages of researchers' data building will use modeling to establish thresholds for materials and architectures, but they will prove those theories with physical tests in toughness, elasticity, and other factors. Not every material can be tested, but the team will verify as many pieces as possible.
Setting materials to music
In addition to creating sets of data for practical use, the team also has devised a creative approach to express those differences. Analysts generally discern the subtle differences in material properties by studying numerical values, charts, and equations. Acar’s group will reimagine those values as musical notes.
To create their symphonies, the team will assign individual notes and chords to the data, which describes common topological curves and shapes. Shapes within similar topologies will produce similar sounds, and subtle changes in the topology can be heard in the translated music as well as seen in the math.
“Symphonies” might be a bit of an overstatement. Acar does not anticipate that translating geometry into music will create the next great classical composition, but she does foresee a different way of evaluating data. When the music is rendered, a student who is more attuned to subtle changes in sound could find a more accessible way to make distinctions in the data.
“Numbers say a lot of things to me, but maybe not to every student,” said Acar. “A song may show more of a comparison, because it’s more understandable and recognizable. These may not be catchy songs, but they will be interesting to hear.”