Nain lab partners with researchers in Japan and Israel to put cancer cells on a tightrope
In studying the composition of cells, a group of researchers has unlocked information that could propel future efforts in understanding the behavior of cancer cells.
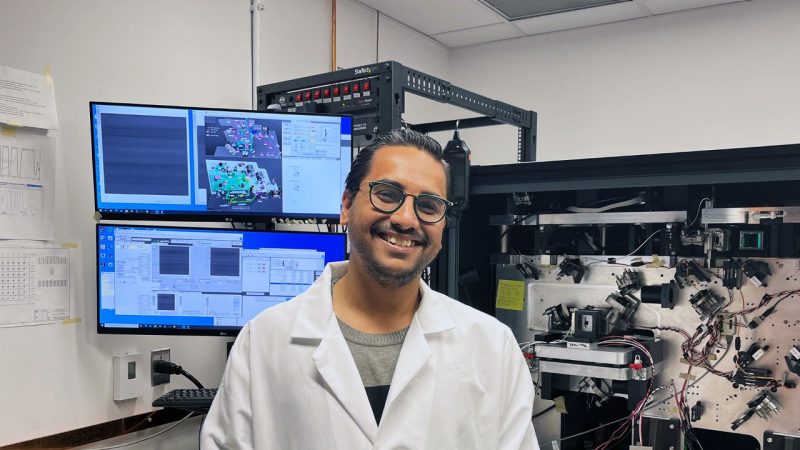
A research group led by Virginia Tech Professor Amrinder Nain and Professor Shiro Suetsugu of the Nara Institute of Science and Technology (NAIST) of Japan has uncovered new findings that explain how cancer cells adhere to their environment. The results of this research were published in the academic journal Advanced Science.
Cellular movement in the body makes life possible, allowing cells to heal wounds, become embryos, and trigger immune defense. Cells move on a network of nanoscale fibers throughout the body, pulling themselves along those fibers in a sort of choreographed micro dance.
To achieve that movement, a cell must be able to adhere to those fibers. This is especially important when a cell is moving on only one fiber, which requires manuevering as deftly as a human tightrope walker. The cell's outside layer, a collection of collagen and fibrous proteins, serves as a scaffold for keeping its grip and migrating.
For this study, the international team of researchers analyzed the cells of glioblastoma and carcinoma, two types of cancer. Nain wanted to more deeply explore how their movement mechanisms work and how the entire cell responds during motion on fibers of different diameters. While he has a deep background in cellular motion, he wanted to take this study much further than he had in the past.
Specifically, he turned his attention to the movement mechanisms themselves. This included studying proteins specific for curvature, and how the whole package works together to propel the soft, malleable cell body. By unraveling the role of curvature-sensing proteins in the tightrope walker's ability to move, researchers can more effectively deploy therapies against harmful cells such as cancer. The team uncovered cell properties that might hold keys to fighting the global killer.
A team from around the globe
Nain contacted Suetsugu because he knew the Japanese researcher’s background in cellular biology. After initial conversations, the two researchers and their graduate students worked through a series of meetings to develop a shared approach to the project. With a clearer view of their objectives, they expanded their team, inviting Virginia Tech colleague and mechanobiologist Bahareh Behkam, biophysicist and theoretician Nir Gov of the Israeli Weizmann Institute of Science, NAIST researcher Tamako Nishimura, and Kyoko Hanawa-Suetsugu of Doshisha University. To experimentally verify theoretical predictions, they also engaged the talents of Yuko Mimori-Kiyouse from the RIKEN Center for Biosystems Dynamics Research.
The graduate student team included Apratim Mukherjee from Nain’s lab, Hooi Ting Hu from Suetsugu’s, and Emanuel Ron from Gov’s. Mukherjee was first author on the published paper.
“Observing this tiny tightrope walker required an interdisciplinary approach, and we were fortunate to recruit a dream team of scientists from across the globe,” said Nain. “This really helped us better understand the choregraphed dance of cellular movement.”
Bringing the cellular pieces together
Nain’s previous work shows how cells move on a single ultra-thin fiber thousands of times smaller than a human hair. His lab has developed techniques to measure single cell forces and has applied them to understanding the inner organization of cellular organelles. Suetsugu’s group has extensively studied the actin protein swirling inside the cell and a family of other proteins labeled IRSp53 that control the shape of the inner cell membrane.
Combining the two bodies of work allowed the team to both observe the movement and interpret the data based on cellular properties. Suetsugu found that proteins play a critical role in the shape of a cell membrane at the edges of cells, and his team used Nain’s research to discover that the actin cytoskeleton system also plays a role in pulling the nucleus of the cell as the entire cell moves on the thin single fiber. This new information hinted to the team that the coordinated movement of the organelles inside the cell affected the way a cell moves.
When the team removed the IRSp53 protein that shapes the inner membrane, something remarkable occurred. The cell diminished actin turnover and the formation of tension-bearing actin stress fibers, causing the cell to contract poorly and become unable to compress its nucleus.
The team wondered if these changes meant the cell would have less ability to move. Normally, cells on a single fiber move in a stick-slip mode that resembles the movement of an inchworm, progressing forward by releasing from its back like a slingshot. During this motion, the nucleus and cell body move together. With the IRSp53 protein gone, the stick-slip motion changed to a slower gliding motion, and the nucleus and cell body motion separated.
Because cellular biology is immensely complex, unlocking the mystery of how cells interact inside the body remains a challenging but significant area of study for the advancement of therapies and drug discovery. Particularly for cancer, the challenge has been discovering ways to stop its growth and spread, which is impacted by cellular movement.
“We are one step closer to discovering how to stop or start cell migration on a single suspended fiber,” said Nain. “Many fundamental questions remain unanswered, but achieving precision in controlling both fiber networks and protein engineering, coupled with new theoretical models and advanced imaging, points to a bright frontier in the multidisciplinary research of tomorrow.”