Researcher receives NIH grant to study biomolecular interactions in hopes of informing drug design
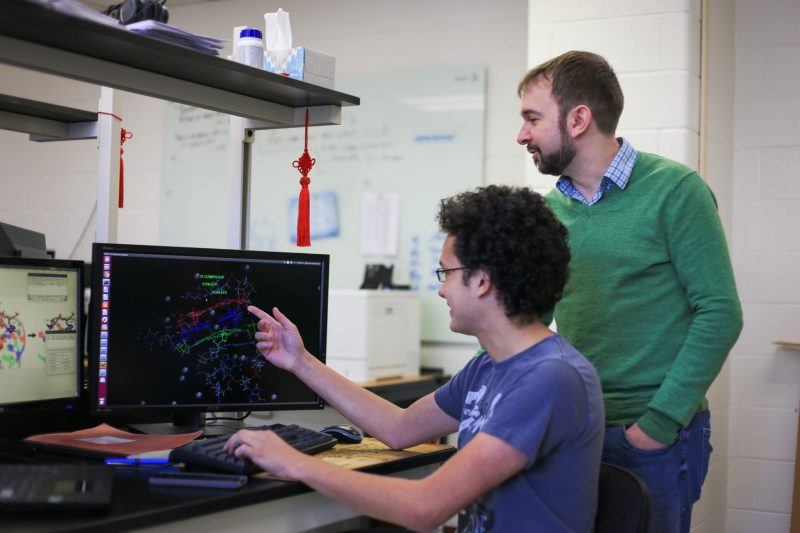
Biomolecules — carbohydrates, proteins, nucleic acids, and lipids — form the foundation of life and are responsible for carrying out every process that occurs in our bodies. Miniscule changes in how these biomolecules fold and interact can lead to severe consequences, such as the development of diseases like Alzheimer’s and Parkinson’s. But, how and why this occurs continues to puzzle scientists.
Justin Lemkul, an assistant professor in the Department of Biochemistry in the College of Agriculture and Life Sciences, received a $1.2 million grant from the National Institutes of Health (NIH) to study how biomolecules fold and interact in an effort to inform better drug design for life-threatening diseases, especially neurodegenerative diseases.
The NIH’s National Institute of General Medical Sciences encourages applicants to submit a “vision for research” instead of specific aims, allowing researchers to address questions holistically instead of in smaller topics. Spread over five years, this unique grant enables Lemkul to study proteins, nucleic acids, and lipids all together under the umbrella of biomolecular folding and interactions.
“All of this work is designed to answer fundamental questions of how biomolecules behave so that we can understand them better for designing drugs against horrible diseases,” said Lemkul, an affiliated faculty member of the Fralin Life Sciences Institute. “That’s sort of what I view my mission in life as being.”
The Lemkul Lab doesn’t look like a typical biochemistry lab — instead of test tubes and beakers, the lab has rows of computers. Lemkul and his team of Ph.D., master’s, and undergraduate students combine computer programming with biochemistry to answer challenging and theoretical questions.
“Computational biochemistry is a somewhat new field, but it is really promising in providing new insights into a lot of human diseases and a lot of fundamental sciences. Harnessing high-performance computing for research is really promising for the future,” said Alexa Salsbury, a third-year Ph.D. candidate. “Looking at ways to integrate computation into research or collaborating with computational researchers can really push the field of scientific research.”
The lab focuses on theoretical approaches to biochemistry and biophysics. Using molecular dynamics simulations, Lemkul and his students study how proteins, nucleic acids, and lipids move and interact with each other to provide insight into how diseases, especially neurodegenerative diseases such as Alzheimer’s and Parkinson’s, might arise.
“Every question in biology is really a question in chemistry, but every chemistry question is really just a physics question. We’re really digging into the details of atoms and electrons. If we can model how these things move around, we can capture all these processes like protein and DNA folding, the nucleic acids’ dynamics, or lipids moving around a whole lot better than anybody ever has before,” Lemkul said.
“If you can understand how the atoms interact with one another and move around, that tells you how life works. So, we try to model that: How do proteins fold? Why do certain proteins unfold in disease states, like Alzheimer’s disease or diabetes? These are all diseases that are linked to a protein doing something bad and then sticking together and interacting with other things in the cell in a negative way.”
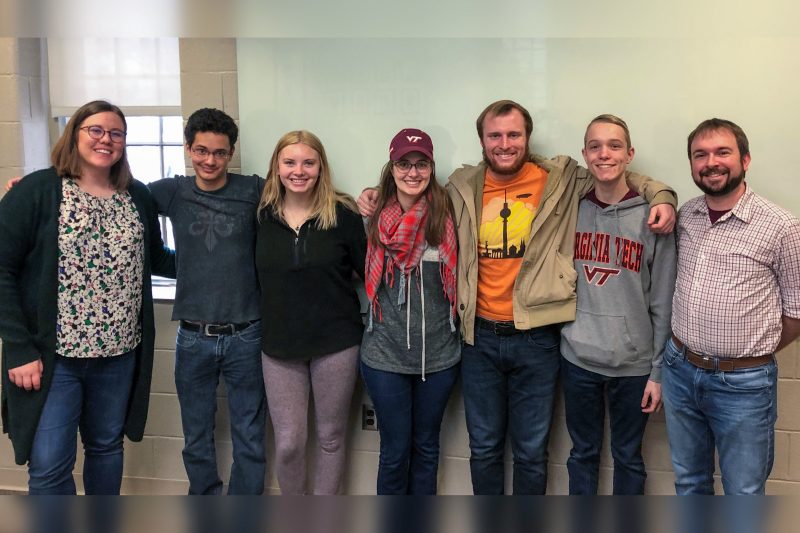
This grant features three separate, but connected, research initiatives. The first project aims to better understand the folding of amyloidogenic proteins, aggregates of proteins that fold into specific forms. When these proteins unfold or are misfolded, they lose the ability to do their job. The amyloid beta-peptide, for example, is linked to the development of Alzheimer’s disease. If a single amino acid in the protein is changed, it can lead to more severe manifestations of the disease such as cerebral hemorrhage and greater memory loss at a much earlier age.
Lemkul studies how the amyloid beta-peptide is involved in the pathology of the disease and why a single amino acid can cause such drastic results. By studying how this happens, Lemkul and his students hope to come closer to figuring out how to prevent it from happening, in theory stopping the development of the disease.
“There’s so much that’s not known about Alzheimer’s disease in general. By studying it systematically, we can get a better idea of how the disease has changed,” said Darcy Davidson, a third-year Ph.D. candidate. “We’re a very small part of a really big puzzle, so if we all work together to get a greater understanding of the disease and hopefully find a cure or treatment.”
The second research project focuses on the formation of G-quadruplexes – highly stable and unique DNA and RNA structures with various conformations that regulate gene expression. When their stability is altered, diseases such as cancer or various neurodegenerative diseases can develop.
G-quadruplexes are targets for drug design because they tell transcription or translation, processes involved with the production of proteins, to turn on or off. For example, cancer is caused by a hyper-expression of a gene that tells cells to keep multiplying. If a drug can destabilize the G-quadruplex that controls gene expression, it will be able to turn that gene off to resume normal cell growth.
Using computational models, the researchers scale down to the nucleic acid level to understand what causes these G-quadruplexes to form and which ions are involved in that process. Drugs and cancer treatments can alter gene expression, but proteins mutate so rapidly that a drug in one patient can be ineffective in another. By identifying which protein is overexpressed, drugs can target the specific region of the protein instead of the protein itself.
“It thrills me to see my students making those discoveries as they watch their simulation outcomes and come up with new ways to analyze them and then they can say, ‘Hey, I figured out why this property of this G-quadruplex is the way it is,’” Lemkul said. “It may seem like a really small thing, but it’s a really big deal! They are small details in the grand scheme of things, but they have big, big impacts.”
The third goal of this research is directly related to computer-aided drug design. Running computer simulations allows the team to study thermodynamic properties and the effects of polarization. These are crucial for developing a drug that can effectively enter a cell and do what it is designed for, like destabilizing a G-quadruplex or preventing an amyloidogenic protein from changing its structure. This type of modeling is largely applicable to studying other diseases, particularly neurodegenerative ones. The ultimate goal of Lemkul’s work is to contribute to drug design that can prevent diseases from developing by going to the source: biomolecules.
“There’s just something really amazing when you see a simulation outcome for the first time. No one else in the world is attacking the same problems that we are. So, my students are the first people to see the things they’re seeing — that’s thrilling,” Lemkul said.
Lemkul completed his undergraduate degree and Ph.D. at Virginia Tech and said that his current work has roots in the undergraduate research he participated in as a student. He now mentors a team of eight students who are all excited about this grant. They are eager to take on new initiatives, travel to prestigious conferences, share the impact of their work, and continue furthering the field of computational biochemistry. As many of them said, “Lemkul is the man.”
— Written by Rasha Aridi