Virginia Tech plays important role in new international neutrino experiment
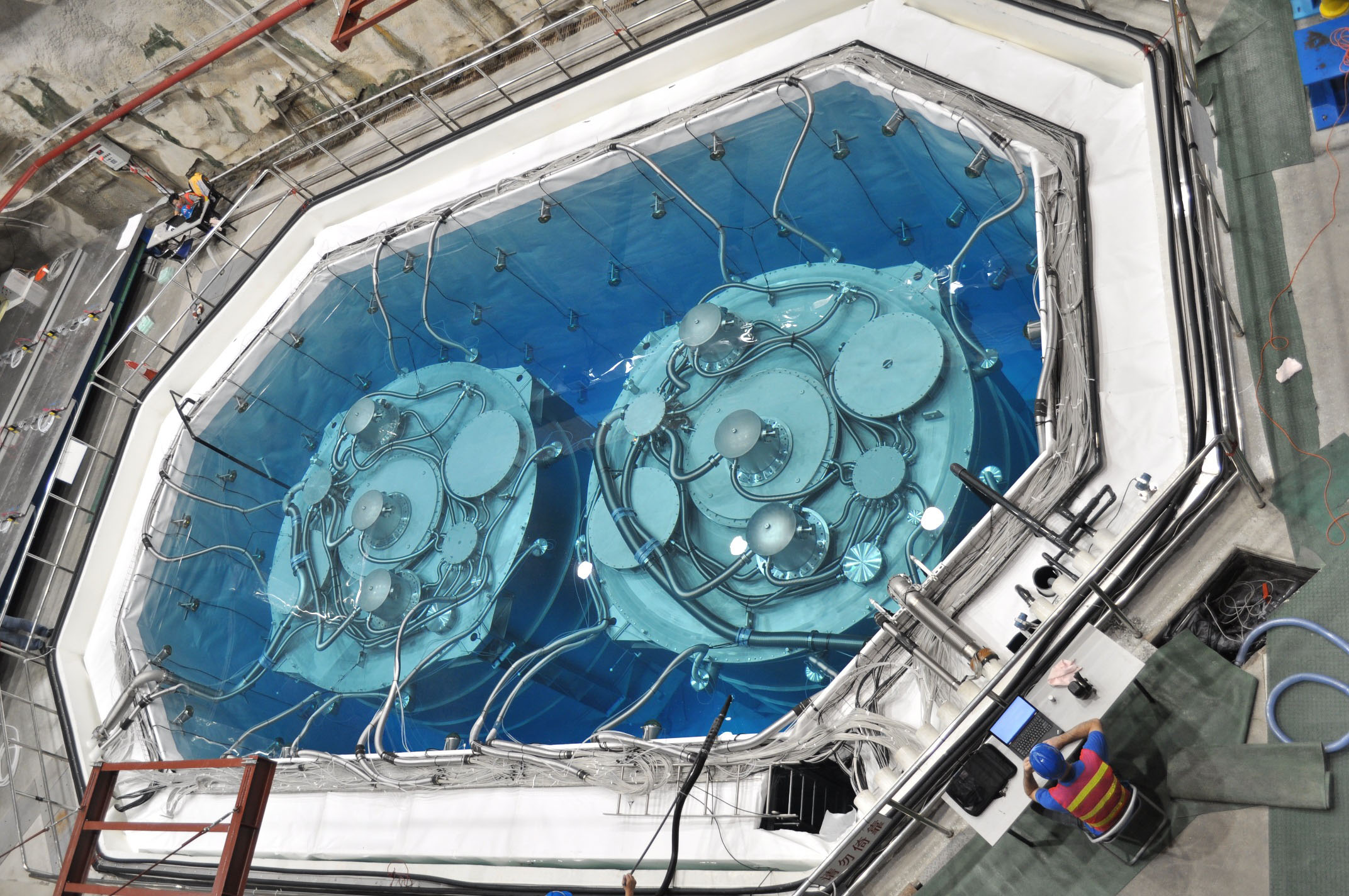
The physics department in the College of Science has been a key player in the Daya Bay Reactor Neutrino Experiment, which recently began its quest to answer some of the most puzzling questions about the elusive elementary particles known as neutrinos.
The experiment’s first completed set of twin detectors is now recording interactions of antineutrinos (antipartners of neutrinos) as they travel away from the powerful reactors of the China Guangdong Nuclear Power Group in southern China.
Neutrinos are uncharged particles produced in nuclear reactions, such as in the sun, by cosmic rays, and in nuclear power plants. They come in three types or “flavors” — electron, muon, and tau neutrinos — that morph, or oscillate, from one form to another, interacting hardly at all as they travel through space and matter, including people, buildings, and planets like Earth.
The start-up of the Daya Bay experiment marks the first step in the international effort of the Daya Bay Collaboration to measure a crucial quantity related to the third type of oscillation, in which the electron-flavored neutrinos morph into the other two flavored neutrinos. This transformation is due to the least-known neutrino “mixing angle,” denoted by θ13 (theta one-three), and could reveal clues leading to an understanding of why matter predominates over antimatter in the universe.
As part of the project, Jonathan Link, associate professor of physics and his team designed and built a high-voltage distribution system for a muon tagging system.
“Muons are a short-lived, heavy cousin to the electron,” Link said. “They are formed when energetic particles slam into the upper atmosphere at nearly the speed of light.
These form showers of particles know as cosmic rays. The muon is the only particle, other than neutrinos, from a cosmic ray however that can make it through any significant amount of earth. Neutrino experiments like Daya Bay are placed deep underground to shield against cosmic rays. The surviving muons must be observed and tagged to prevent them from being confused for neutrinos.
Link and his team also played a significant role in the detector’s calibration systems. They built a custom system for making short light pulses at several locations throughout the detector’s water pool. These light pulses are used to calibrate the light sensitive detectors, which can identify the individual particles of light made when a muon passes through the water.
“One of the most striking characteristics of our universe is that it is made up of matter, as opposed to antimatter,” Link said. “But everything we know about the Big Bang says that it must have consisted of equal parts matter and antimatter. So where did the antimatter go?”
Link said many physicists now believe that in the earliest moments after the Big Bang, neutrinos played a crucial role in creating this asymmetry through a slight difference in the way they interact with matter and antimatter.
“This slight difference may have been enough to create a small excess of matter over antimatter,” Link said. “Over time, only this small excess remained,” Link said. “Life on earth is only possible because of this matter-antimatter asymmetry. Experiments like Daya Bay will go a long way to demonstrate if this model is valid.”
Nearly 40 institutions from around the world are involved in the Daya Bay Collaboration. The experiment will be carried out over the course of 4 years. Link’s team included: Professors Leo Piilonen and Patrick Huber, Research Assistant Professor Debabrata Mohapatra, graduate students Joseph Hor and Meng Yue, and Research Specialist Jo Ellen Naron.